Microbiological Specifications in Food Operations
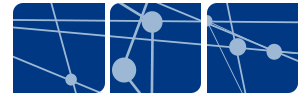
Introduction
Microbiological specifications are tools used to help manage risk in a food business. A specification defines the limits of acceptable and unacceptable conditions (from a microbiological perspective) at important decision points in the manufacturing and distribution continuum, from ingredient specifications to final product specifications. Unfortunately, specifications receive most attention when an analytical result is “out of specification” (OOS) and quick decisions are required, possibly with limited information and much at stake. In these circumstances most of the attention goes to “the number” or other result that was set as a limit, with little attention paid to the broader context of how and why the specification was created in the first place.
In this white paper we will explore the types, uses, and creation of microbiological specifications. This paper should be considered merely an introduction to a topic on which thousands of pages of statistics and opinions have been written over the years.
It is important to note that microbiological specifications and testing do not, alone, ensure food safety or quality. Their primary role is to verify that other elements of the management process are under control such that hazards are being appropriately mitigated. Moreover, microbiological specifications are not the only type of specification that will be in place for any given food commodity.
What do we mean by “specification”?
Specifications are one category of criteria that define limits for particular purposes:
- Standards are mandatory legal or regulatory criteria specified by government to protect public health.
- Specifications are agreed criteria between buyers and producers that define product quality and safety attributes required by the buyer.
- Guidelines are advisory criteria about acceptable or expected microbial levels.
However, in common usage, all three types of criteria may be referred to as specifications. There is often little differentiation between guidelines and specifications. Both are primarily “private” tools internal to an organization, between parties to a commercial agreement or recommended by bodies with a common interest such as trade associations. Failure to adhere to standards may trigger mandatory recalls, or criminal penalties. Failure to adhere to specifications and guidelines may also be costly through commercial penalties or civil liability.
Elements of a specification
To be effective, a specification must identify certain key elements:
- The matrix (product, ingredient, or environmental sample) to be tested.
- The microbiological target or targets (microorganism, toxin, or population of concern).
- The method or methods to be used.
- The sampling plan.
- The acceptable limit for each microbiological target.
When developing the sampling plan and setting acceptable limits, it is immediately apparent that pathogens with a low infectious dose and severe disease potential should have more stringent limits than microorganisms primarily of quality concern. In addition, the target
consumer, product characteristics and intended use should be considered. Foods for infants or immunocompromised populations may have more stringent limits than foods for the general population. Foods in which microbial populations may grow may have more stringent limits than
those in which microbial populations will stay the same or decline. Foods considered ready to eat are likely to have more stringent limits than products intended for reheating or cooking. In this way we strike a balance between protecting consumers and managing the cost of testing.
The specification may also identify the action to be taken if one or more of the microbiological targets is outside the acceptable range. An OOS result defines the matrix as not acceptable for the intended purpose. In other words, it is “rejected”. However, that rejection can mean diversion to some other purpose where the reason for rejection can be addressed. For example, if a lot of raw meat tests positive for Salmonella, it could be diverted from a retail stream to a production stream heading for a cooking process that provides a kill step. At the other end of the scale, if a production lot of probiotics has a lower count than expected, it could be diverted to use in a product with a lower label claim or dosed at a higher rate to ensure the expected activity in the final product.
Matrix
Within the laboratory, anything that we test is considered a matrix, whether that be product, ingredient, environmental swab or sponge, or portion of unidentified material. The matrix matters because it will have a historical association with a characteristic microbial ecology. What pathogens have been linked to the matrix? If it is a food product or ingredient, how does it spoil? What is a normal microflora when it is produced, handled, and stored under good conditions? A matrix may also be associated with a particular intended use. Is it for retail sale or further processing? How will risks associated with it change during handling? If it is an environmental sample, is it for pathogen detection or for a more general indication of sanitation effectiveness? The answers to these and related questions will help to identify suitable microbiological targets.
Microbiological target or targets
A microbiological specification should include microorganisms and/or microbial toxins that tell us that the normal operation is not in control or is moving out of control. Sometimes that will be a pathogen, e.g., Salmonella or Listeria monocytogenes. Sometimes it will be an indicator
5 of sanitary conditions, e.g., coliform count. Sometimes an indicator of overall microbiological condition, such as total aerobic plate count, will give the most useful picture. In all cases, selection of the microbiological target should be based on knowledge of the matrix, its intended use, and its place in the overall food chain. Targets should be chosen based on association with safety or quality issues, especially those that are controlled by appropriate processing and handling practices and those significant to the intended consumer.
Method or methods to be used
For any particular microbiological target there could be several different test methods, all with a demonstrated ability to detect or enumerate the microorganism of interest. However, differences between methods might lead to differences in results, particularly in marginal cases. For example, U.S. regulatory agencies favor incubation of pathogens at 35 °C, whereas much of the rest of the world favors 37 °C for the same purpose. The difference is small, but if the pathogen grows faster at 37 °C than at 35 °C there could be circumstances where the population is just below the limit of detection after incubation at 35 °C and just above it after incubation at 37 °C. The reverse may also be possible if the background flora grows faster at 37 °C and suppresses the growth of the target pathogen. There can be many other slight differences between methods contributing to the potential for disputes between customer and vendor, or between laboratories using different methods. For these reasons, it is important to state clearly the method with which adherence to the acceptable limit is to be assessed. In an effective specification, the microbiological method will be known to be fit for purpose through method validation or matrix verification.
Sampling plan and acceptable limits
The sampling plan is at the heart of a specification, defining the number and size of samples to test as well as the associated limits. For products and ingredients, sampling plans are applied to a “lot”, which is a quantity processed in such a manner that all portions of that lot are expected to be similar. Lot sizes can vary dramatically, but in a manufacturing operation, the lot would be expected to be no larger than the amount of product made between two clean breaks. The plans specify required sampling and define limits on test results that determine acceptability of the lot. There are different approaches to creating sampling plans,
considered below.
Attributes Sampling Plans
Most microbiological sampling plans are “attributes” plans, which were widely promoted in the 1970s by the International Commission on Microbiological Specifications for Foods (ICMSF) (8). In attributes plans, the microbiological test results are used to attribute the sample to one
of a number of classes. A two-class plan has “acceptable” and “defective” classes. A three-class plan has “acceptable”, “marginally acceptable”, and “defective” classes. Attributes plans lose some of the information associated with continuous data, such as plate count results, because every result is merely assigned to a class and all the results in that class carry exactly the same weight. For example, it does not matter if results in the “marginally acceptable” class are at the bottom or the top of the marginally acceptable range. Attributes plans work well when knowledge of the history of the matrix or the distribution of microorganisms within it is incomplete or absent. They can be used in situations where results could be continuous, e.g., colony count data, or binary, e.g., “detected” versus “not detected” results for pathogens.
The high-level principle of an attributes plan requires the definition of the number of samples tested (n), the marginally (m) and maximum (M) acceptable limits, and the number of samples (c) permitted to exceed the marginal limits. No samples are permitted to exceed the maximum defined limits without triggering the rejection of the lot. However, rejection of the lot may allow for diversion of the product or ingredient for other uses and/or reprocessing. Key elements of attributes plans can be found summarized in Table 1.
Table 1. Summary of the elements of an attributes sampling plan.
Element |
Definition |
c |
The number of samples permitted to exceed m. |
m |
The marginally acceptable limit defined by good manufacturing practice. |
M |
The maximum acceptable limit defined by safety. |
n |
The number of samples tested |
2-class |
Results fall into one of only two classes – acceptable or unacceptable |
3-class |
Results may fall into one of three classes – acceptable, marginally acceptable, or unacceptable. Once the number of marginally acceptable results exceeds c, the lot is considered defective. |
Sample |
A portion of a lot taken for analysis |
Test portion |
That part of the sample actually analyzed |
Composite |
A blend of a stated number of test portions analyzed together in a single test. |
There are two broad types of attributes sampling plan:
- 3-class plans, in which results may fall into one of three classes – acceptable, marginally acceptable, or Once the number of results in the marginally acceptable class (values above m) exceeds c, the lot is considered defective.
- 2-class plans, in which results fall into one of only two classes – acceptable or Since there is no marginally acceptable class, c = 0.
Plan stringency
Plans are constructed to become more stringent as the risk associated with the microbiological target increases. In this context, the elements of risk are the severity of illness, the potential number of people exposed and/or ease of person-to-person transmission, and the vulnerability of the target consumer. Examples of how these elements can be combined leading to different risk levels include the following:
- The lowest overall risk would be from a spoilage microorganism in a product that does not support its growth. There is a potential for economic loss but no food safety risk.
- An intermediate risk, and the lowest food safety risk, would be from a pathogen which, at high concentrations, causes “only” a mild illness via a toxin formed in the food, but the pathogen cannot grow, or dies, during storage and distribution, and the product is made in small lots for limited geographical distribution, to a normal, healthy adult consumer. Here there is no risk of person to person spread, the exposed population is limited, and the consequences are unpleasant but not, usually, dangerous.
- The highest risk would be from a pathogen that causes a severe disease with high potential mortality or morbidity and the potential for person-to-person transmission from a low infectious dose, in a product that supports growth of the pathogen, with large number of servings per lot, wide geographic distribution and a vulnerable consumer such as infants or persons with compromised immune systems. In this case, the exposed population is large and vulnerable, and the consequences are potentially deadly.
Plans with larger values of n and smaller values of c, m and M are more stringent. Table 2 illustrates the relationship between the risk associated with the microbiological target and the amount of sampling recommended encompassing fifteen cases from lowest risk (Case 1) to highest risk (Case 15).
Table 2. Relationship between the risks associated with a food product and stringency of attributes sampling plan design.
Health hazard |
Effect of conditions of food handling on degree of concern and case Reduce No change Increase |
||
None, effect on shelf-life |
Case 1 3-class, n = 5, c = 3 |
Case 2 3-class, n = 5, c = 2 |
Case 3 3-class, n = 5, c = 1 |
Low, indirect |
Case 4 3-class, n = 5, c = 3 |
Case 5 3-class, n = 5, c = 2 |
Case 6 3-class, n = 5, c = 1 |
Moderate, direct, limited spread |
Case 7 3-class, n = 5, c = 2 |
Case 8 3-class, n = 5, c = 1 |
Case 9 3-class, n = 10, c = 1 |
Moderate, direct, extensive spread |
Case 10 2-class, n = 5, c = 0 |
Case 12 2-class, n = 10, c = 0 |
Case 12 2-class, n = 20, c = 0 |
Severe, direct |
Case 13 2-class, n = 15, c = 0 |
Case 14 2-class, n = 30, c = 0 |
Case 15 2-class, n = 60, c = 0 |
Sampling plan effectiveness
The effectiveness of any particular sampling plan is illustrated by the plan’s operating characteristics (OC) curve, which shows the probability of accepting a lot of product with different defect rates. Figure 1 compares the OC curves for two 2-class plans. The plan on the left, n = 60, c = 0 is about 95 % likely to reject a lot with a 5 % defect rate. It is appropriate for a severe risk use case. The plan on the right, n = 5, c = 2 has a high probability of accepting a lot with only a 5 % defect rate and becomes 95 % likely to reject the lot only when the defect rate approaches 80 %. Thus, it is appropriate for cases where the risk of either a safety or quality consequence is low.
Notice, also, that the steepness of the OC curve increases with the number of samples tested. A steep curve makes the cutoff between an acceptable and an unacceptable lot very clear. Both parties to a transaction would like the differentiation of an acceptable from an unacceptable lot to be as clear as possible, but the cost of testing often discourages testing large numbers of samples. We all like to save money but in this case, we should recognize that in agreeing to test fewer samples, both parties to a transaction agree to accept more risk. The vendor has increased the risk of rejection of a good lot entailing lost revenue, potentially investigational expenses, and damaged reputation. The purchaser has increased the risk of acceptance of a defective lot and any consequences that may then ensue.
Figure 1. Comparison of operating characteristics curves for two 2-class sampling plans. The n = 60, c = 0 curve on the left is steeper, giving better separation of acceptable from unacceptable lots, and rejects lots with a smaller proportion of defective units than the n = 5, c = 0 curve on the right.
One practice, which is common but unfortunate, is to test only one sample to make a decision. Effectively this creates a sampling plan with n = 1. This approaches the power of a plan with n > 1 only at very low defect rates, where both plans accept almost everything, or at very high defect rates where both plans reject almost everything. Unfortunately, we cannot compensate for reducing the number of samples by increasing the size of the test portion (sample actually subjected to testing). For example, a sampling plan of n = 15 with a 25 g test portion could be handled by testing a 375 g composite sample. However, this provides much more protection to both parties than a sampling plan of n = 1 with a 375 g test portion, even though in both cases the total test portion is the same. The reason for this is explained below in “Why do these plans test multiple samples?”
Acceptable limits
The acceptable limit is the other component of sampling plan stringency. This is the quantity that gets most of the attention. How is it determined?
Remember that attributes sampling plans assume no prior knowledge of the microbiological condition of the lot. This means that acceptable limits are set in relation to what we know about the risks associated with different microbiological targets.
For infectious pathogens such as Salmonella or Escherichia coli O157:H7, where consumption of only a few cells may cause illness, we would set the acceptable limit as close to zero as practicable. For most purposes, this level is “not detectable in 25 g”, or some multiple thereof. This limit would be applied with a 2-class sampling plan with no marginally acceptable class (c = 0). The number of samples to be tested can range from n = 5 to n = 60 depending on the overall risk profile, with n increasing as risk increases. These situations would be similar to those illustrated in the bottom two rows of Table 2.
For Listeria monocytogenes, the infectious dose appears to be higher than for the pathogens above. However, the regulatory authorities in the U.S. maintain a “zero tolerance” policy towards this microorganism in ready-to-eat foods, so here, too, the acceptable limit is “not detectable in 25 g” or some multiple thereof, again using a 2-class sampling plan with c = 0. Some other countries have chosen a low limit of < 100 colony forming units (cfu)/g for detectable Listeria at the end of product shelf life. We will not examine the complexities of these choices here and encourage readers to explore further if those circumstances may apply to them.
Toxigenic pathogens such as Bacillus cereus and Staphylococcus aureus generally only cause harm when they grow to levels that allow them to form toxins in the food. We certainly want to maintain a good margin of safety, but we might be able to tolerate small populations in a few marginal samples. These microorganisms might have a marginally acceptable level m of 100 cfu/g in 2 of 5 samples tested, and an unacceptable limit of 1,000 cfu/g. This is specified using a 3-class plan where n = 5, c = 2, m = 100 cfu/g and M = 1,000 cfu/g and is illustrated in the second and third rows of Table 2. Indicators of poor handling following thermal processing, such as Enterobacteriaceae count or coliform count may have a low limit such as with a 3-class n = 5, c = 2 plan with m = 10 and M = 100 cfu or most probable number (MPN) per g or mL as seen in the second row of Table 2.
Spoilage microorganisms and general quality indicators, for example lactobacilli or aerobic plate count may have greater limits as with a 3-class plan of n = 5, c = 3, m = 100 and M = 10,000 cfu/g. This is illustrated in the first row of Table 2.
There are many sources for guidance on appropriate acceptable limits for many microorganisms and matrices e.g. (4, 7, 8, 11, 12, 15, 21). Most of these have been presented with a view to protecting public health and without specific knowledge of any particular company’s product, process, customer, etc. Hence, it is our responsibility to select and/or adapt the guidance to our particular situation. We may choose to use more stringent specifications to protect quality, provided that all parties to a transaction are agreeable. Equally, we may choose to use less stringent specifications if we have other controls in place that will ensure equivalent product safety. If we are unable to find guidance specific to our circumstances, we can conservatively adapt from scientifically sound sources including those cited above. However, we must also be aware of regulatory standards that do not permit a less stringent interpretation, such as those pertaining to infant formula (18).
Why do these plans test multiple samples?
One of the difficulties in microbiological testing is that the target microorganism is usually not uniformly distributed. Complicating matters further when we are concerned about pathogens, their population could be very small. In pathogen testing, we typically expect to detect a single microorganism in a 25 g sample (or some multiple of 25 g). It is easy to see that our chances of finding a microorganism present at such low population levels is increased if we test more samples. It is less intuitive, but nevertheless true, that our chances of success are greater if we take 15 samples, each of 25 g from across the lot than if we take the same total amount as a single sample of 375 g from just one location. Figure 2 illustrates this conceptually. The relationship is not simple, varies with level of contamination, and can require significantly larger total sample sizes to give equivalent protection with only a single sample than with a larger number of smaller samples. Unfortunately, the food manufacturing industry is reluctant to test a large number of samples due to cost, which must be passed onto customers, potentially resulting in an uncompetitive market position.
When we are dealing with quantitative data, such as aerobic plate counts or other indicators of quality, it is easy to fall into the trap of accepting a lot with a count that is only a little bit greater than the specification limit “just this once”, especially with a result based on only a single sample. One easily overlooked benefit of a multiple sample plan is that there is no room for a discussion about a result being “only a little bit OOS”. Once the result is greater than the limit m for more than c samples, or if any samples exceed M the lot is not acceptable.
Figure 2. Sampling for non-uniformly distributed microorganisms. Note that the total sample size (area inside the circles) is the same in both (a) and (b), but the chance of finding the contamination increases in (b) because more locations within the lot are sampled.
Examples
Examples are shown in Table 3, indicating changing stringency with concern and use case. As we progress down the table, we will move to progressively less stringent cases based on the risk assessments indicated in the references. This table is presented as an illustration of the approach without an opinion on the relative merits of the sources.
The most stringent case (Case 15) is applied to beef trim at the packing operation. Sixty 6.25 g samples per lot are composited into a single 375 g test portion for detection of pathogenic Shiga toxin producing E. coli (STEC) (20). If the lot tests positive, it is diverted from retail ground beef to a process with a kill step (3). This very stringent specification is used because STEC can cause severe disease, and ground beef production gives the opportunity for comingling to produce large amounts of product with highly dispersed contamination and the potential for contamination to increase if the product is temperature-abused. Note that the industry is largely moving away from this n = 60 approach to surface sampling of larger portions of the lot using a MicroTally™ cloth.
As we move down Table 3, we see progressively less risky use cases. Infant formula is intended for a susceptible population, so specifications for Salmonella and Cronobacter are quite stringent since both can cause severe disease. However, the hazard from Cronobacter is largely confined to the infant, whereas Salmonella may also infect a parent or other carer, hence the specification for Salmonella is more stringent than that for Cronobacter.
Continuing to move down Table 3, we pass through examples of a toxigenic pathogen (B. cereus), which needs to grow to large numbers to cause illness, indicators of potential presence of enteric pathogens or generally poor sanitary practices (coliforms), and finally onto quality indicators (APC). At each step, the specification becomes less stringent as the risk reduces.
Table 3. Examples of microbiological specifications illustrating how they vary according to purpose. These are all intended to be illustrative and should not be taken as recommendations.
Matrix |
Consumer |
Concern |
Target |
Risk Case |
Method |
Sample (g) |
n |
c |
m |
M |
Source |
Beef trim |
Ground beef production |
Pathogen |
STEC |
15 |
USDA MLG Ch. 5C.01 |
6.25 |
60 |
0 |
0 |
- |
(19) |
Infant formula |
Infants |
Pathogen |
Salmonella |
14 |
FDA BAM Ch. 5 |
25 |
30 |
0 |
0 |
0 |
(18) |
Infant formula |
Infants |
Pathogen |
Cronobacter |
13 |
FDA BAM Ch. 29 |
10 |
15 |
0 |
0 |
0 |
(18) |
Frozen cooked rice |
General |
Pathogen |
B. cereus |
8 |
FDA BAM Ch. 14 |
50 |
5 |
1 |
103 |
104 |
(8,9) |
Dried milk |
General |
Indicator |
Coliforms |
5 |
FDA BAM Ch. 4 |
50 |
5 |
1 |
10 |
100 |
(8,9) |
Dried milk |
General |
Quality |
APC |
2 |
FDA BAM Ch. 3 |
50 |
5 |
2 |
104 |
105 |
(8,9) |
Frozen fish |
General, to cook |
Quality |
APC |
1 |
FDA BAM Ch. 3 |
50 |
5 |
3 |
5 x 105 |
107 |
(8,9) |
By the time we get to the last row of Table 3, looking at aerobic plate count in frozen raw fish intended for cooking by a general consumer, we are interested only in quality and have a very lenient Case 1 sampling plan, with an M so high that the product is likely close to spoilage.
Variables Sampling Plans
When we are concerned about spoilage organisms, or pathogens where we can afford to find small numbers, such as Bacillus cereus, we could choose to use a variables plan. Variables plans can be more cost-effective because they use all the information from testing including both the absolute value of test results and the observed variance of those results, whereas attributes plans lose all the variance data and keep only the quantitative determination of “acceptable”, “marginal”, or “unacceptable” for each sample. However, to design a variables sampling plan we need to know the distribution of the target microorganism in the matrix. Moreover, if a test lot of matrix presents with greater variability than was used to set the decision rules, then the plan will not perform as expected.
Determining the distribution of the target microorganism in the matrix takes a great deal of sampling and statistical analysis. For practical purposes, the distribution of microorganisms in foods and food ingredients is often, but not always, lognormal (13) and the mean and standard deviation of the logarithm of counts when the process is operating normally can be used to define the distribution. Furthermore, the variability of counts in a lot from a process that is out of control is likely to be considerably higher than the variability when the process is in control. For these reasons, it is unusual to find microbiological specifications set using variables plans. Rather than provide an extensive description of an approach that is not commonly used with microbiological testing, the reader is referred to other sources (10, 14).
A Simplified Approach
Recognizing that the industry will frequently default to n = 1 sampling plans on cost grounds, we can ask if a simplified approach that tests only a single sample has any value. Indeed, it can, when using specifications within a program to verify that a process or operation is running under control, and delivering results as expected. For this purpose, we can develop an internal specification based on our understanding of “normal” operation. It is quite common in food manufacturing to run a multi-shift review of product quality during initial startup of a new product. Collecting quantitative microbiological data during this period can establish a baseline on which to build a specification. For example, aerobic plate count, Enterobacteriaceae count, or mold and yeast count could all serve for this purpose. Determine the range of counts achieved during normal operation by taking the results from 20-60 shifts and calculating the mean and standard deviation of the log counts. This can be done easily in a spreadsheet program. If we set the acceptable limit at two standard deviations above the mean, 97.5 % of all production from future runs should be below this limit when the process or operation remains under control. An action to investigate results above this level is likely to discover some loss of control that must be corrected. Results greater than three standard deviations above the mean almost certainly mean that the process or operation is out of control since 99.85 % of all results should be less than this level. This simplified approach works because the operation is under our control and the microbiological result is just one piece of information telling us about its safety. In the section on “Trend analysis”, we will discuss ways to get more value from these data. Note that testing only a single sample per lot using a qualitative test for a pathogen will find only the grossest of excursions out of control.
What about environmental samples?
Most of this white paper has been concerned with specifications for food products or ingredients. However, a large portion of all microbiological testing in food safety management is done on sponges, swabs or other samples taken as part of an environmental monitoring program intended to demonstrate the effectiveness of plant sanitation. For these samples, acceptable limits are set to trigger appropriate actions when operations are out of control or moving out of control, but the lot concept does not apply. Instead, it is common to divide food production environments into zones:
- Zone 1 – food contact
- Zone 2 – areas immediately adjacent to zone
- Zone 3 – surfaces adjacent to zone 2 within the production space, g., floors, walls, drains, cleaning tools, fork-lift trucks, etc.
- Zone 4 – areas outside the production space, g., hallways, break rooms, locker rooms, etc.
Environmental sampling does not usually follow a statistical sampling plan, instead covering the facility such that more attention is paid to areas with greater opportunities to act as sources of contamination (5, 12). Specifications for routine environmental samples should be based on what can normally be achieved when the operation is under control, with data for indicator populations collected and analyzed as suggested in “A Simplified Approach”, above, for products. It is also useful to understand the baseline prevalence of pathogens in environmental samples but as a general rule, detection of foodborne pathogens in the processing environment should trigger some remedial action or investigation.
How do specifications differ for beneficial microorganisms?
Beneficial microorganisms offer some desirable functionality. In foods and dietary supplements, the classic beneficial microorganisms are probiotics. The United Nations Food and Agricultural Organization and the World Health Organization define probiotics as “live microorganisms, which, when administered in adequate amounts, confer a health benefit on the host” (6). For such beneficial microorganisms, the specification may have separate components for food safety management, e.g., absence of pathogens in a specified sample size, and quality management, e.g., minimum concentration of the beneficial microorganism. One selling point of probiotic products (and a required labeling element) is the amount of beneficial organisms in the product. The US Food and Drug Administration requires the label to state the probiotic content in mg and permits it also to be shown in numerical form as colony forming units, per g or per serving. Most probiotic products are labeled with a claim about the number of beneficial microorganisms “billions of probiotics per serving” or similar.
Since the beneficial microorganisms are typically a key active ingredient, they are clearly present and should be uniformly distributed. Thus, we can use a specification that ensures presence of the claimed number of microorganisms at the end of shelf-life. Spore-based probiotics are typically stable if appropriately packaged. Vegetative probiotics such as lactic acid bacteria and bifidobacteria tend to decline in viability slowly over time so usually require adding some overage at the point of manufacture to allow for this decline.
The simplified approach above should work well for verifying that the operation is producing concentrations or amounts of the intended beneficial microorganisms at the expected levels. Samples from 20-50 production lots stored to the end of shelf-life and enumerated before and after storage can generate the mean and standard deviation of results at the beginning and end of shelf-life. To ensure that production is in control, the specification could then be set, for example, two standard deviations below the mean and product would be packaged with sufficient overage to achieve the dose claimed on the label at the end of shelf life. The size of this overage should be based on process variability and method variability. Of these, method variability is usually significantly larger than process variability. Sampling plans and acceptable limits for pathogens and indicators in a probiotic product are likely to be similar to the limits for other products with a similar target consumer and overall risk profile. However, when testing for pathogens or indicator microorganisms in probiotic preparations, significant modifications to standard methods might be required to overcome the competitive pressure from the probiotic microorganisms and verification of method performance with the probiotic matrix is highly recommended.
How to get more value from your data
Trend analysis
It is common practice to review microbiological results on receipt. Provided that they are within the established specification, no action is taken beyond filing them away. This is unfortunate because the compiled results from multiple lots have additional value. A simple plot of quantitative results over time can show if results are trending up or down. Both trends should be investigated. Results trending up might indicate a process heading out of control that can be corrected before an OOS result occurs. Trends heading down might indicate an unrecognized process improvement that can be identified and formalized. More sophisticated trend analysis using a control chart could use several different rules to indicate a process heading out of control and allow corrections to be made at an early stage. Descriptions of common rules and a free control chart template are available from the American Society of Quality (1).
Reducing process variability
When considering a sequence of results from an ongoing operation, simple statistical analysis can tell us something about the distribution of results. For example, microbiological results are frequently lognormally distributed. In other words, the logarithms of the individual results will follow a normal distribution (the classic “bell curve”) when gathered together. We can further describe this distribution with the mean and standard deviation. The mean tells us the “center point” and the standard deviation describes the “width” of the bell, which indicates how variable the results are. This variability in a sequence of results from a process or manufacturing operation can be used to estimate the frequency of future extreme events (16, 17). It is not possible to predict when such extreme events will occur but results from processes or operations with high variability are more likely to generate extreme values than those with lower variability. Hence, any action that reduces process variability also reduces the likelihood of an outbreak of food poisoning or a product recall, even if the connection is not immediately obvious. Perhaps of more immediate benefit, a reduction of process variability can reduce the incidence of OOS results and their associated headaches. These actions are outside the scope of this article but covered in detail in publications on statistical process control and quality management (2).
Closing remarks
Microbiological specifications serve primarily to verify that food manufacturing processes are under control and provide the safety and quality required by the customer and consumer. For this reason, specifications should be set with reference to objective measures of risk relevant to the matrix, the intended consumer, and the point in the process at which the testing is being done. There are many sources of information that will suggest upper bounds of acceptable limits for many microbiological targets e.g. (4, 7, 8, 11, 12, 15, 21), and these can serve as a guide when initially setting specifications. There are also good sources of advice for sampling plan design. A good specification including a well-designed sampling plan can help to maximize the safety and quality benefits obtained from the dollars spent on microbiological testing.
Out of specification results always cause concern. A well-run laboratory will have many controls in place to ensure accuracy of results, as well as traceability of actions on a sample, and a process to investigate laboratory operations associated with an OOS result. However, the laboratory usually has no say in defining the specification. A poorly designed specification runs the risk of failing to provide the protection expected, or of triggering an OOS result for a normal condition. Probably the largest contributor to both of these risks is the tendency to build a specification around the result from a single sample.
Where to get help
Eurofins Food Assurance consultants can help you to work through any individual circumstances to construct an appropriate microbiological specification for your needs.
Acknowledgments
Colleagues Andrzej Benkowski, Chris Crowe, Dan DeMarco, and Doug Marshall reviewed and offered helpful comments on this article.
References
- American Society of Control Chart. Available at: https://asq.org/quality-resources/control-chart Accessed 19 April, 2023.
- American Society of What Is Statistical Process Control? Available at: https://asq.org/quality-resources/statistical-process-control Accessed 20 April, 2023.
- AMI Foundation. The Role of an N-60 Sampling Program in Ground Beef Safety. Available at: https://www.meatinstitute.org/index.php?ht=a/GetDocumentAction/i/55845 Accessed 19 April, 2023.
- Bolton, E, et. al. 2009. Guidelines for Assessing the Microbiological Safety of Ready-to-Eat Foods. London: Health Protection Agency, https://assets.publishing.service.gov.uk/government/uploads/system/uploads/attachment_data/file/363146/Guidelines_for_assessing_the_microbiological_safety_of_ready- to-eat_foods_on_the_market.pdf Accessed 5 April, 2022.
- Environmental Monitoring Program. Eurofins White Paper. Available at: https://marketing.eurofinsus.com/acton/attachment/25929/f-0822/1/-/-/-/-/Environmental_Monitoring_Program_White_Paper.pdf Accessed 25 April, 2023.
- FAO/WHO. Evaluation of health and nutritional properties of probiotics in food, including powder milk with live lactic acid bacteria. Food and Agriculture Organization of the United Nations and World Health Organization Expert Consultation Report.
- Food Standards Australia New 2016. Compendium of Microbiological Criteria for Food. https://www.foodstandards.gov.au/publications/Documents/Compendium_revised%20Dec%202022.pdf - Accessed 5 April, 2022
- International Commission on Microbiological Specifications for Foods (ICMSF) 1974 Microorganisms in Foods 2 Sampling for Microbiological Analysis: Principles and Specific Applications, 2nd Edition, Blackwell Scientific Publications
- International Commission on Microbiological Specifications for Foods (ICMSF) 1978 Microorganisms in Foods 1: Their Significance and Methods of Enumeration, 2nd Edition. University of Toronto Press, Toronto, Canada.
- International Commission on Microbiological Specifications for 2002. Microorganisms in foods 7. Microbiological Testing In Food Safety Management. Edited by R.B. Tompkin et al. Kluwer Academic/Plenum Publishers, New York.
- International Commission on Microbiological Specifications for Foods (ICMSF) 2011 Microorganisms in Foods 8 Use of Data for Assessing Process Control and Product Acceptance, edited by K. M. J. Swanson, et al. Springer Science+Business Media, New York, NY, USA.
- International Commission on Microbiological Specifications for Foods (ICMSF) Microorganisms in Foods 7 Microbiological Testing in Food Safety Management, 2nd edition, edited by R. L. Buchanan, et al. Springer International Publishing, Cham, Switzerland.
- Kilsby, C., and M.E. Pugh. 1981. The Relevance of the Distribution of Micro-organisms Within Batches of Food to the Control of Microbiological Hazards from Foods. Journal of Applied Bacteriology, 51, 345-354.
- Legan, D and M.M Vandeven. 2003. Sampling Techniques. In: Detecting Pathogens in Foods, edited by T.A. McMeekin. Woodhead Publishing Ltd. Cambridge, England.
- National Advisory Committee on Microbiological Criteria for 2018. Response to Questions Posed by the Department of Defense Regarding Microbiological Criteria as Indicators of Process Control or Insanitary Conditions. Journal of Food Protection, 81:115-141
- Nussinovitch, and Peleg, M. (2000) Analysis of the fluctuating patterns of microbial counts in frozen industrial food products. Food Research International, 33, 53-62.
- Peleg, (2002) Interpretation of the irregularly fluctuating microbial counts in commercial dairy products. International Dairy Journal, 12, 255-262.
- United States Code of Federal Regulations 21 CFR 106.55 Controls to prevent adulteration from microorganisms. Available at: https://www.ecfr.gov/current/title-21/chapter-I/subchapter-B/part-106/subpart-B/section-106.55 Accessed 12 May, 2023.
- United States Department of Agriculture Food Safety Inspection 2014. FSIS Compliance Guideline for Establishments Sampling Beef Trimmings for Shiga Toxin- Producing Escherichia coli (STEC) Organisms or Virulence Markers. Available at: https://www.fsis.usda.gov/sites/default/files/import/Compliance-Guide-Est-Sampling-STEC.pdf Accessed 19 April, 2023.
- United States Department of Agriculture Food Safety Inspection Service 2023. DIRECTIVE 10,010.1 Rev.5 Sampling Verification Activities For Shiga Toxin-Producing Escherichia coli (STEC) in Raw Beef Products https://www.fsis.usda.gov/sites/default/files/media_file/2020-07/10010.1.pdf Accessed 19 April, 2023.
- United States Pharmacopeia. 2023. General Chapter <2023> Microbiological Attributes of Nonsterile Nutritional and Dietary Supplements. USP-NF. Rockville, MD: United States Pharmacopeia.
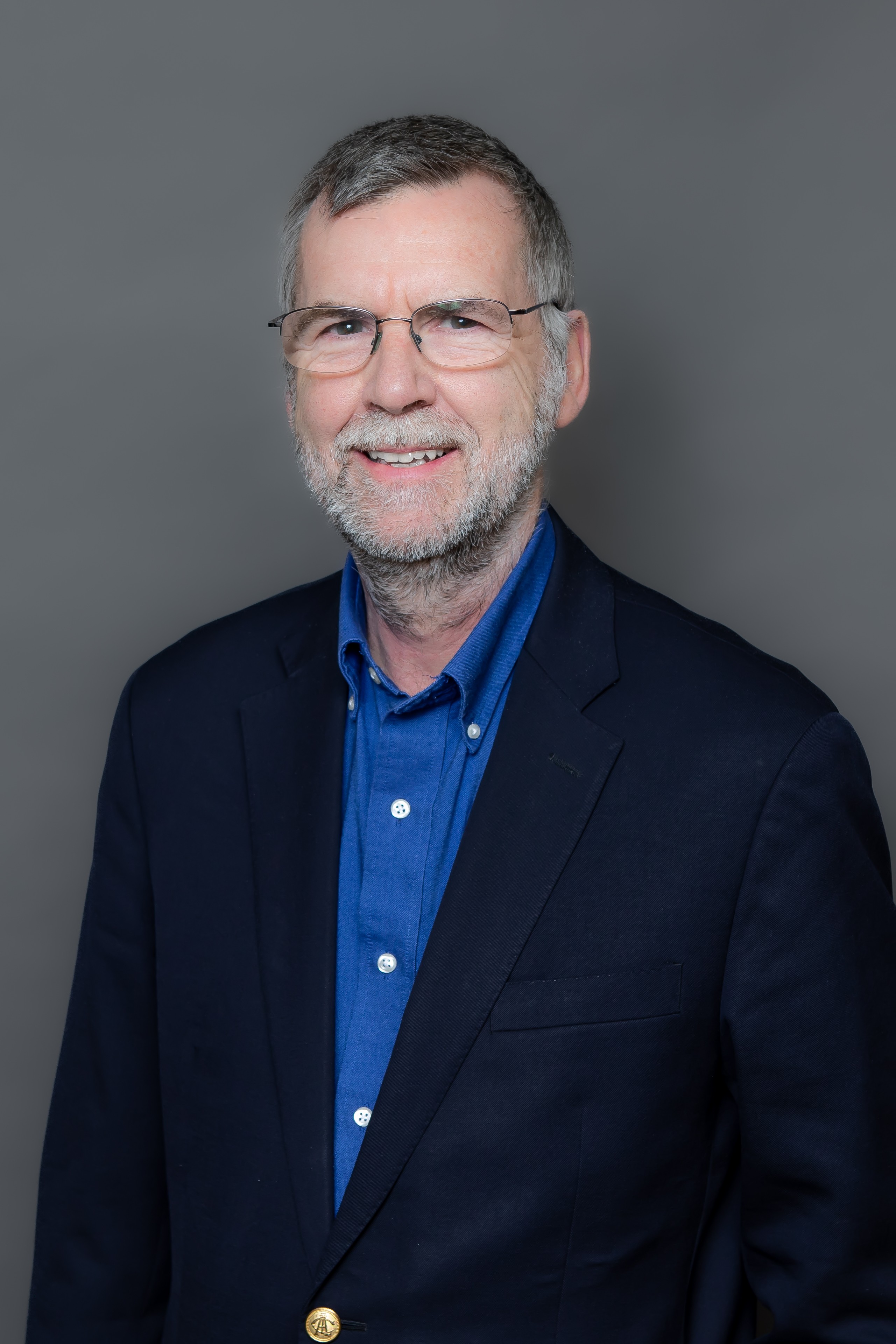
The Author:
J. David Legan, PhD
Scientific Director, Eurofins Microbiology Laboratories