Microbiological Shelf-life and Product Spoilage: Achieving Objectives and Investigating Failures
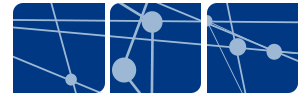
Introduction
Product spoilage rarely makes the headlines but can still be very costly for a producer in terms of lost product, damaged reputation, and loss of future sales. Prevention is the best approach to avoid these costs but if prevention fails, we must investigate, take corrective action, and improve controls to prevent reoccurrence.
This white paper will provide a brief overview of both prevention and investigation of spoilage. By focusing on principles, it will not be able to answer every question, but rather will illustrate questions to ask during product design and failure investigations.
Designing for shelf-life
The shelf-life of a food, ingredient, or dietary supplement is a function of many different factors. Together they determine what kind of spoilage occurs, and how quickly that spoilage is likely to happen. This has been taught in food microbiology as the “hurdle concept” since 1976 (10) and imagines that each factor is a hurdle to microbial growth. Together, multiple hurdles combine to limit the occurrence of microbiological spoilage within a defined period of time.
In this paper, we will consider only microbiological spoilage, but products may also spoil through physical changes such as loss of texture or acceptable appearance, or chemistry-driven organoleptic changes such as development of rancidity. This paper is likely to be more relevant to “manufactured” products that have undergone more than minimal processing than to minimally processed foods such as produce or raw meat, where shelf-life is very short. When we get to the sections on diagnosing spoilage, it will be important to remember that microbiological activity can generate many metabolites with strong odors. These might mislead us into suspecting chemical adulteration, hence it will be prudent to keep an open mind. First, let us review the factors that determine product shelf-life.
Intrinsic Factors
Intrinsic factors are those that result from the product formulation, and generally are related to the desired sensory properties of the product. In most cases, intrinsic factors can be modified to extend shelf-life, but only within a limited range of values before the desired product sensory qualities are sacrificed.
Water activity
Water activity (aw) is a measure of the “availability” of water. The definition of aw is “the vapor pressure of water surrounding the product in a sealed system, divided by the vapor pressure of pure water at the same temperature”. It is expressed as a decimal, e.g., 0.97. Sometimes, instruments measure the equilibrium relative humidity (ERH) around the product in a sealed chamber and express it as a percentage. It is easy to convert between these two quantities because ERH = aw x 100. Anything dissolved in water will lower its vapor pressure and since food products usually contain a blend of water and other ingredients, it is the other ingredients that cause a reduction in aw.
Table 1: Minimum aw for growth of selected microorganisms. Modified from Fontana, 2007 (7).
Water Activity |
Bacteria |
Molds |
Yeasts |
0.97 |
Clostridium botulinum E Pseudomonas fluorescens |
|
|
0.95 |
Escherichia coli Clostridium perfringens Salmonella spp. Vibrio cholerae |
|
|
0.94 |
Clostridium botulinum A, B Vibrio parahaemolyticus |
Stachybotrys chartarum |
|
0.93 |
Bacillus cereus |
Rhizopus stolonifer |
|
0.92 |
Listeria monocytogenes |
|
|
0.91 |
Bacillus subtilis |
|
|
0.90 |
Staphylococcus aureus (anaerobic) |
Trichothecium roseum |
Saccharomyces cerevisiae |
0.88 |
|
|
Candida |
0.86 |
Staphylococcus aureus (aerobic) |
|
|
0.85 |
|
Aspergillus clavatus |
|
0.84 |
|
Byssochlamys nivea |
|
0.83 |
|
Penicillium expansum |
Debaromyces hansenii |
0.80 |
|
Penicillium citrinum |
Zygosaccharomyces bailii |
0.78 |
|
Aspergillus flavus |
|
0.75 |
|
Aspergillus restrictus Aspergillus candidus |
|
0.71 |
|
Eurotium chevalieri |
|
0.70 |
|
Aspergillus amstelodami |
|
0.62 |
|
|
Zygosaccharomyces rouxii |
0.61 |
|
Xeromyces bisporus |
|
<0.60 |
- - - - - - - - - - - - No microbial growth - - - - - - - - - - - - - - - - - - - - - - |
||
*Mold and yeast names current according to Species Fungorum (2) |
Water activity matters because as it becomes lower, the number of different microorganisms able to grow reduces (7) (Table 1) and the growth rate of those microorganisms becomes slower. Hence, lower aw translates into reduced spoilage risk and increased microbiological shelf-life, as well as changes in the potential spoilage microflora. Traditional preservation techniques of drying and salting work by reducing aw. Once aw drops below 0.6, foods are protected from microbiological spoilage indefinitely, provided that aw does not increase again for any reason. Table 2 shows the ranges of aw values in selected food examples.
Table 2: Examples of typical aw ranges for food items. Modified from Schmidt et al, 2020 (18).
CATEGORY: Subcategory; Item(s) |
Low aw |
High aw |
Dairy: milk, fresh; whole, 2%, fat free, 1.5%, skim. |
0.988 |
0.996 |
Fruits, fresh: limes, plums, apricots, nectarines, oranges, apples, peaches, melon, strawberries |
0.980 |
0.997 |
Meat, raw: pork, lamb, ground beef, |
0.980 |
0.992 |
Dairy: yogurt; various manufacturers and flavors. |
0.973 |
0.988 |
Beverages, ready- to-drink: margarita mix; branded carbonated beverages, regular and diet. |
0.972 |
0.999 |
Meat and poultry, ready to eat: ham, smoked turkey, corned beef (canned), meat paste (canned) |
0.971 |
0.984 |
Fruits: fruit juices; grape, apple, cherry, orange, tomato, pineapple |
0.970 |
0.990 |
Vegetables, fresh: onions, green beans, parsnips, Brussels sprouts, carrots, asparagus, cucumbers, spinach |
0.970 |
0.988 |
Fish, canned: tuna, sardines, mackerel, salmon. |
0.968 |
0.974 |
Dairy, cheese, fresh: Cheddar, Swiss |
0.950 |
0.960 |
Dairy, cheese, processed. |
0.910 |
0.946 |
Baked goods: bread; multiple brands and varieties. |
0.900 |
0.970 |
Jams, jellies, preserves: grape, peach, raspberry, strawberry |
0.802 |
0.852 |
Fruits: fruit juice concentrate; orange |
0.800 |
n.a. |
Baked Goods: cake; multiple brands and varieties |
0.720 |
0.945 |
Dairy: cheese, fresh; parmesan |
0.693 |
0.881 |
Meat: dried; beef jerky, salami, pepperoni |
0.691 |
0.860 |
Fruits: dried; raisins |
0.510 |
0.540 |
Breakfast cereals: multiple brands and varieties |
0.157 |
0.472 |
Cereal grains, legumes, nuts and seed. |
0.147 |
0.750 |
Milk: dried* |
0.137 |
0.277 |
Baked Goods: cookies and crackers; multiple brands and varieties |
0.112 |
0.649 |
*Outlier recorded at aw = 0.75, in line with dairy protein powders. |
|
|
pH
The pH of a material is a measure of acidity or alkalinity on a scale from 0 to 14, where 0 is the most acidic, 14 is the most alkaline and 7.0 is neutral. Most foods are neutral to acidic (Table 3). Similar to aw, the more extreme the pH value becomes, the fewer microorganisms are able to grow (Table 4), and the slower the growth rate of those that can. The traditional preservation techniques of lactic acid fermentation and pickling work by acidifying the product and reducing the pH.
Table 3: Typical pH of common foods. Adapted from extension sources (1, 13).
Item |
Low pH |
High pH |
Item |
Low pH |
High pH |
Eggs, whole, frozen |
8.5 |
9.5 |
Beef (ground) |
5.1 |
6.2 |
Cake, chocolate |
7.2 |
7.6 |
Sugar |
5.0 |
6.0 |
Egg solids, whole |
7.1 |
7.9 |
Molasses |
5.0 |
5.5 |
Egg whites |
7.0 |
9.0 |
Oysters |
4.8 |
6.3 |
Crackers |
7.0 |
8.5 |
Peppers |
4.7 |
5.5 |
Freshwater fish (most) |
6.9 |
7.3 |
Eggplant |
4.5 |
5.3 |
Cake, pound |
6.8 |
7.1 |
Tomatoes |
4.3 |
4.9 |
Shrimp |
6.8 |
7.0 |
Tomatoes, puree |
4.3 |
4.5 |
Fish (most fresh) |
6.6 |
6.8 |
Cucumbers, pickled |
4.2 |
4.6 |
Egg solids, whites |
6.5 |
7.5 |
Mayonnaise |
4.2 |
4.5 |
Chicken |
6.5 |
6.7 |
Tomatoes, juice |
4.1 |
4.6 |
Broccoli |
6.3 |
6.9 |
Corn starch |
4.0 |
7.0 |
Avocados |
6.3 |
6.6 |
Ketchup |
3.9 |
3.9 |
Milk |
6.2 |
7.3 |
Cherries, Royal Ann |
3.8 |
3.8 |
Butter |
6.1 |
6.4 |
Olives, green fermented |
3.6 |
4.6 |
Herring |
6.1 |
6.4 |
Plums, Red |
3.6 |
4.3 |
Olives, black |
6.0 |
7.0 |
Tomatoes, paste |
3.5 |
4.7 |
Brussels sprout |
6.0 |
6.3 |
Mangoes, green |
3.4 |
4.8 |
Flour |
6.0 |
6.3 |
Apricots |
3.3 |
4.8 |
Ham |
5.9 |
6.1 |
Orange juice |
3.3 |
4.2 |
Carrots |
5.9 |
6.4 |
Peaches |
3.3 |
4.1 |
Mangoes, ripe |
5.8 |
6.0 |
Apple, eating |
3.3 |
4.0 |
Squash, yellow, cooked |
5.8 |
6.0 |
Pineapple |
3.2 |
4.0 |
Beans |
5.6 |
6.5 |
Cucumbers, dill pickles |
3.2 |
3.7 |
Spinach |
5.5 |
6.8 |
Rhubarb |
3.1 |
3.4 |
Lamb |
5.4 |
6.7 |
Strawberries |
3.0 |
3.9 |
Potatoes |
5.4 |
5.9 |
Grapefruit |
3.0 |
3.8 |
Pork |
5.3 |
6.9 |
Grapes, seedless |
2.9 |
3.8 |
Beets |
5.3 |
6.6 |
Plums, Blue |
2.8 |
3.4 |
Bread |
5.3 |
5.8 |
Ginger ale |
2.0 |
4.0 |
Tuna fish |
5.2 |
6.1 |
Vinegar |
2.0 |
3.4 |
Papaya |
5.2 |
6.0 |
Lemon juice |
2.0 |
2.6 |
Table 4: pH ranges for growth of different groups of microorganisms. Modified from Lund & Eklund, 2000 (12).
Species name |
Minimum pH |
Optimum pH |
Maximum pH |
Bacteria (most) |
4.5 |
6.5-7.5 |
9.0 |
Acetobacter spp. |
3.0 |
5.0-6.0 |
|
Alicyclobacillus |
2.0 |
3.5-4.0 |
7.0 |
Alicyclobacillus acidocaldarius |
2.5 |
3.4-4.0 |
5.5 |
Enterobacter agglomerans |
3.6 |
|
|
Escherichia coli (non-pathogenic) |
4.0 |
|
|
Clostridium butyricum |
|
|
|
Gluconobacter spp. |
3.0 |
5.5-6.0 |
|
Lactobacillus spp. |
2.9 |
|
6.3-6.8 |
Pathogenic bacteria |
|
|
|
Bacillus cereus |
5.0 |
6.0-7.0 |
8.8 |
Clostridium botulinum |
4.6 |
|
|
Clostridium perfringens |
5.0 |
|
9.0 |
Salmonella spp. |
3.8 |
7.0-7.5 |
9.5 |
Yeasts (general range) |
1.5-3.5 |
4.5-6.8 |
8.0-8.9 |
Candida krusei |
1.3 |
|
|
Saccharomyces cerevisiae |
1.6 |
|
|
Pichia membranifaciens |
1.9 |
|
|
Zygosaccharomyces bailii |
2.2-2.5 |
|
>7.0 |
Molds (general range) |
1.5-3.5 |
4.5-6.8 |
8.0-11.0 |
Moniliella acetoabutans |
<3.3 |
|
|
Aspergillus flavus, A. parasiticus, A. niger |
2.0 |
5.0-8.0 |
>11.0 |
Fusarium equiseti |
<3.3 |
5.0-8.0 |
>10.4 |
Penicillium verrucosum |
<2.1 |
6.0-7.0 |
>10.0 |
Preservatives and other microbial inhibitors
Many food components have some antimicrobial activity that goes beyond their effect on aw or pH. Organic acids, such as acetic, lactic, and citric acids, are often important components of the flavor system of the product through their acidifying action. Others such as sorbic, propionic and benzoic acids are primarily added as antimicrobials. In all cases, these acids become more antimicrobial as the pH drops, with this effect being in addition to any reduction in microbial growth attributable solely to the pH value. Other additives including alcohols, nitrites or sulfites have utility in particular applications. Many of these ingredients and additives are regulated, and specific restrictions can be found in the Substances Added to Food database (22) from FDA formerly known as EAFUS (Everything Added to Foods in the United States). Whether these compounds are derived naturally, or “artificially” makes no difference to their activity but does affect the way that they are shown on product labels because consumers have, for decades, expressed a preference for “natural” ingredients. Hence, product labels may show ingredients such as “cultured corn sugar”, which contains lactic acid and/or sodium lactate, or “cultured celery juice,” which contains sodium nitrite as a “friendlier” or “cleaner” alternative to artificial preservatives.
Structure
Food structure can significantly affect shelf-life by affecting where and how microorganisms can grow, and the distribution of nutritive and inhibitory factors. For example, butter is a water-in-fat emulsion where individual water droplets are so small that only a small amount of growth can occur in any individual droplet before it is “full,” and the microbial growth is physically constrained. Salted butter contains about 11-17% water and 1.0-1.6% salt (median values 16.1% and 1.3% respectively) (19). However, the salt partitions into the water droplets, so that the concentration of salt on water is around 8% and the range of aw is from 0.83 to 0.95 (18), which constrains most bacterial growth.
Extrinsic factors
Extrinsic factors are those pertaining to the environment around the product. We have great capacity to manipulate extrinsic factors to our advantage but cost or product factors may constrain our ability to maximize their effect on shelf-life.
Temperature
Probably the first factor that comes to mind for most of us is temperature. We all have personal experience of food keeping for longer under refrigeration than at room temperature, and for very long periods when it is frozen. All microorganisms have an optimum temperature range for growth. For most food spoilage microbes that optimum temperature range is around 20-40 °C (68-104 °F). As temperature moves away from that range, either higher or lower, growth rates are reduced and the number of different microorganisms able to grow becomes smaller. Principles for use of temperature for safe storage of foods have been developed over many years and the current view of the US Department of Agriculture is that for safety, foods should be stored below 40 °F (4.4 °C) or, if being kept hot for serving, above 140 °F (60 °C) (21). Further, for safety, foods should be cooled from processing temperatures to below 70 °F within two hours and to below 40 °F within a further two hours.
The shelf-life of foods held above 140 °F is a matter of hours at most, and spoilage is through some degradation of taste, texture, appearance, or other quality attributes. We will not consider this temperature range further. Foods stored below 40 °F but not frozen may have a shelf-life of days to weeks or months, depending on the intrinsic factors of the product and its microflora. If foods are frozen and stored at 0 °F (-18 °C) or lower, they may last for months or years and spoilage is through physical and/or chemical changes.
Atmosphere
The atmosphere immediately surrounding the food can affect both the type and rate of microbiological growth that can occur. We can extend the shelf-life of many foods by choosing to remove or modify the atmosphere within the package.
Foods with no internal spaces can be vacuum-packed, in which all the air is removed before the package is sealed. This leaves the residual oxygen concentration at extremely low levels, effectively limiting the growth of aerobic microorganisms such as molds and many bacteria and promoting an anaerobic spoilage microflora that can grow in the absence of oxygen. Most yeasts and many bacteria can grow anaerobically, though to cause spoilage these anaerobes must also be able to tolerate the product’s intrinsic factors and the storage temperature.
Vacuum packaging will crush any product with internal voids, including many bakery items, or foods with soft textures or soft components. For these foods, we can replace some or all of the air in the package with a custom gas blend. Such blends often contain carbon dioxide (CO2) and nitrogen or other inert gases. The CO2 is actively antimicrobial but tends to dissolve into any water present in the food. The inert gas helps to exclude oxygen while protecting the package from collapsing as the CO2 dissolves.
Packaging barrier properties
The most obvious function of packaging is to protect products against gross contamination and physical damage, but it does much more than that. For long shelf-life items, the moisture-barrier properties prevent products from drying out in low humidity environments and from absorbing moisture from high humidity atmospheres. If some form of modified atmosphere is used, whether vacuum packaging or use of a replacement gas mixture, the oxygen and CO2 barrier properties are also important. Glass and metal containers have the highest barrier properties (and highest cost and weight). Plastic structures cover a wide range of barrier properties. In general, for higher barrier properties plastics require laminated structures and are more expensive than those with low barrier properties. Pinhole leaks and poor seals can compromise the performance of any package and cause loss of any expected shelf-life enhancement from the use of a modified atmosphere. Selection of appropriate packaging involves a tradeoff of cost, weight, convenience, and performance.
Processing
In the context of this whitepaper, we are concerned with the ability of food processing to provide a treatment lethal to microorganisms that could otherwise sicken consumers or spoil the product. However, it is important that whatever process we select does not adversely affect the desired product qualities of taste, texture, and appearance. To simplify matters we can think of “sterilizing” processes that destroy all viable microorganisms, pasteurizing processes that destroy vegetative microbial cells but not bacterial spores and non-lethal treatments that redistribute microorganisms but have no lethal effects. Process lethality is a function of temperature and time, in the case of thermal processing.
Canning is the classical example of a sterilizing process. A canning process delivers commercial sterility, which is defined in the US Code of Federal Regulations (4) as “…the condition achieved -
- By the application of heat which renders the food free of -
- Microorganisms capable of reproducing in the food under normal nonrefrigerated conditions of storage and distribution; and
- Viable microorganisms (including spores) of public health significance; or
- By the control of water activity and the application of heat, which renders the food free of microorganisms capable of reproducing in the food under normal nonrefrigerated conditions of storage and distribution. “
Other, less lethal, thermal processes kill some fraction of the vegetative microorganisms present but not any bacterial spores, thereby reducing the starting population and extending the time until the population grows large enough to cause spoilage. When applied deliberately for this purpose, or to improve safety, we call these pasteurization processes. However, the same effect can be achieved when the thermal process is used to cook the food to achieve desired functional and organoleptic properties.
Processes that redistribute microorganisms can have either positive or negative effects on microbiological shelf-life but rarely in a manner that can be exploited.
Lethal processes commonly use heat, but several non-thermal lethal processes such as high pressure, pulsed electric field, ultraviolet light, ionizing radiation and treatment with antimicrobial gases, are all used in limited applications.
Microbial factors
If microorganisms present can grow given the intrinsic and extrinsic factors discussed above, we will often see characteristic spoilage patterns, for example:
- Low aw products tend to have mold spoilage if solid or yeast spoilage if
- High aw, neutral or nearly neutral products tend to exhibit bacterial
- High aw, acidic products tend to show mold and/or yeast spoilage but a limited range of bacterial spoilage is also possible.
- In vacuum-packaged products, anaerobic bacteria or yeasts tend to dominate spoilage and will often, though not always, produce gas leading to visible pack bloating and the condition known as “blown pack spoilage.”
Realistic expectations
The interplay of all the factors described to this point determine the shelf-life and spoilage population in any particular product. After understanding the intrinsic and extrinsic factors and processing pertaining to a product, an experienced food microbiologist will be able to assess the most probable characteristics of spoilage. They will also likely be able to estimate shelf-life. However, there are so many variables at play, that it is wise to confirm an accurate shelf-life through stability and challenge studies. The key point to remember is that every product spoils eventually if not consumed first. In exceptional cases, this could take several decades (6, 11) and not all spoilage is caused by microorganisms. If failure to achieve the intended shelf-life is because of microbiological spoilage, and within a timescale that is reasonable or typical for the product given the factors discussed above, then the underlying problem may be an unrealistic expectation of the achievable shelf-life, possibly based on incomplete information.
Investigating the failure
The Swiss cheese conceptual model is used in many areas of failure analysis (16, 17). It sees every barrier to failure (in this case spoilage) as imperfect – like a slice of Swiss cheese with holes. It is illustrated in Figure 1 showing that failure occurs if the holes in all the layers align. This model helps us to remember that when a product spoils more quickly than was expected, an investigation to determine the root cause must ask many questions and make many observations. Only then will we have the information to determine steps needed to correct the problem and prevent it from reoccurring.
Figure 1: "Swiss cheese" model of product failure. Each slice represents an incomplete barrier. When all barriers are breached, a spoilage event (failure) can occur.
Symptoms
Observations of symptoms are the most logical place to begin.
- Appearance: What does the product look like?
- Bubbly? (Or pack bloating)?
- Slimy?
- Moldy? (Mold spores are readily inhaled and may trigger allergies or infections so we should not deliberately smell a moldy product.)
- Smell: If it’s not visibly moldy, what does it smell like?
- Alcoholic / yeasty?
- Sour?
- Putrid?
- Rancid / paint-like?
- Rotten eggs?
- Dirty feet?
- Taste: We will not deliberately taste a spoiled product but sometimes spoilage is discovered when spoiled food is inadvertently eaten, and that information can provide insights.
Product, package, and distribution
Next, we can examine the intrinsic and extrinsic factors discussed above looking for conditions that permit the observed spoilage symptoms, for example:
- What is the aw? If the product has multiple components, is aw uniform across all of them?
- What is the pH? Do all components of the product have the same pH (if relevant)?
- What is the product form: solid, liquid, emulsion, multi-component. And where is the spoilage physically appearing? In one component? At the junction between two components?
- What are the product’s ingredients? Does the ingredients list include a preservative?
- Is the package damaged? If yes, is that common to all spoiled units?
- If the product is vacuum- or modified-atmosphere packed, what are the oxygen, CO2 and moisture barrier properties?
- At what temperature is the product stored, distributed and sold?
- Are we certain that the product experienced no temperature-abuse?
- If not, what was the temperature and time history of the product?
Diagnosis: microbiological testing
The answers to the questions above may be enough to determine the cause of spoilage, but more commonly they suggest microbiological tests that would shed more light on the problem. For example,
- Common causes of pack bloating, bubbling, or other forms of gassy spoilage include yeasts, coliforms, heterotrophic lactic acid bacteria and Clostridium
- Slime formation is often caused by some species of Leuconostoc, Pseudomonas, or Bacillus.
- Sour notes can be caused by acid-producing bacteria including Lactic acid bacteria, Enterobacteriaceae, or some species of Bacillus or Clostridium.
- Putrid notes are caused by microorganisms capable of breaking down proteins including some Pseudomonas and Clostridium
- Rancid notes are often the result of purely chemical oxidative processes but can be caused by bacteria including some Pseudomonas and Clostridium species, some yeasts, and many Some microbial enzymes are robust enough to survive thermal processing and accelerate chemical processes in otherwise shelf-stable, commercially sterile products.
This is not an exhaustive list but intended to illustrate that symptoms can help us to design a rational testing approach for diagnosis of the cause of the spoilage. At this point, standard methods are not always adequate because they were not designed for this purpose, so sometimes we must consider method modifications or even alternative methods. It is always best, if possible, to run any tests alongside the same tests run on an unspoiled product since the comparison will highlight differences between the two. A few hypothetical examples will illustrate the thought process but should not be considered an exhaustive list.
Example 1
A vacuum packed, refrigerated, ready-to-eat cooked meat product (high aw, near-neutral pH) with an expected shelf-life of 8 weeks presents after only 4 weeks with an expanded package indicating gas production. On aseptically opening the package, a sour odor is noticed. This suggests the presence of gas-producing microorganisms. We hypothesize the presence of heterofermentative lactic acid bacteria from the smell. We will test for heterofermentative lactic acid bacteria and, from the same sample, Enterobacteriaceae, yeasts and anaerobic spore formers. All tests will be run at the “standard” incubation temperature for said test, and with incubation at a non-standard 15-20 °C in case the spoilage organism is only able to grow at low temperatures. In this example, all the tests are quantitative methods or “counts”. Running all tests in parallel means that we do not lose time or sample if our initial hypothesis is wrong.
We find very high counts of heterofermentative lactic acid bacteria, with Enterobacteriaceae, yeasts and anaerobic spore formers all below the limit of detection. These results indicate lactic acid bacteria as the immediate cause of the gas production in the package. Since lactic acid bacteria are not particularly heat resistant, hence would not have survived a validated and correctly operated cook step, the root cause investigation must confirm correct operation of the cook step and investigate potential sources of cross-contamination during slicing and packaging.
Example 2
A fruit juice product, subjected to an ultra-heat treatment (UHT) process, aseptically filled into cartons, and distributed at ambient temperature, presents with a medicinal odor when opened. There is no distortion of the carton to indicate gas production. The aw is high, and the pH is low. The spoilage observed strongly suggests that outgrowth of Alicyclobacillus spores was the cause, however we need to eliminate the possibility of other common spoilers of fruit juice including yeasts, lactic acid bacteria and acetic acid bacteria. None of these three groups are heat-resistant, hence we would not expect them to be present in this product. If we did find them, their presence would point to either a process failure or an issue with post-process recontamination. Since this product is distributed at ambient temperature, there is no need to consider incubation at reduced temperatures.
In this case, testing reveals a high count of Alicyclobacillus. Moreover, the Alicyclobacillus test demonstrates that the strain present can produce guaiacol, which is the direct cause of the medicinal odor. Since Alicyclobacillus is a spore former it may have survived the UHT process and further investigation should look at the condition of raw materials, the sanitation of processing equipment, and both the validation and correct operation of the thermal process.
Example 3
Retail packages of raisins arrive at the laboratory with a note indicating that there is a white bloom on the surface of many of the individual fruits. There is no indication that the packages are bloating or damaged. Upon opening a package there is no noticeable odor other than that of the raisins themselves and when we aseptically sample a few of the fruits into an empty Petri dish there is a low, white bloom, on the wrinkled surface of the raisins. Examination under a low powered stereomicroscope reveals that the white bloom is slightly furry and, therefore, most likely a mold. Since the mold is visible, there is little point in a standard “mold count” and an alternative method can be employed. One option is to place contaminated raisins directly on the surface of a convenient medium for growing molds. Since the raisins have a low aw, we place additional raisins on the surface of a medium with a very high (50%) glucose concentration to support growth of molds that prefer to grow at lower aw. Plates are incubated upright at 25, 30 and 35 °C and are reviewed every 3 days until there is clear mold growth present. We also take samples from each package for aw measurement.
After a few days we see the beginnings of white growth across the surface of the high-glucose medium and we have results for water activity of the raisins. The measured value ranged from 0.67 to 0.72 across the samples tested. We have demonstrated that the white bloom was evidence of mold growing on the raisins. We also have a measured aw, which is unusually high for this product and suggests that either the raisins were not completely dried, or they encountered moisture at some time after drying. Further investigation should examine the drying process and downstream opportunities for moisture ingress.
Diagnosis: microbiome analysis
One of the more useful modern tools for spoilage diagnosis is the ability to characterize the entire microbiome of a spoiled sample using Next Generation Sequencing (NGS) technologies. These technologies have dramatically increased the speed, and cut the cost of sequencing, thereby offering diagnostic capability that was cost-prohibitive only a few years ago. If applied to DNA extracted directly from spoiled and unspoiled product, NGS can quickly indicate important differences between the two, including qualitative differences in the identities of any microorganisms present, and semi-quantitative differences in the relative abundance of those microorganisms. However, NGS cannot show whether any organisms identified are alive or dead, or the absolute numbers present. Hence, NGS is ideally used to supplement or guide cultural approaches. Figure 2 illustrates the ability of microbiome analysis to show differences in microbial populations. It shows the major components of the microbiome of cold smoked salmon, before and after aerobic incubation at 4 °C for 30 days. The population changed from one dominated by Carnobacterium species to one dominated by Brochothrix thermosphacta, a lactic acid bacterium common in spoilage of chilled raw meats. The plots are simplified from supplementary data of Jarvis et al (8).
Figure 2: Microbiome comparison: relative abundance of dominant microorganisms on cold-smoked salmon before and after 30 days’ aerobic incubation at 4 °C. The population, initially dominated by Carnobacterium, became dominated by Brochothrix species. Pseudomonas, Enterococcus, Lactococcus, and Serratia species were also present. Data from Jarvis et al, 2022 (8).
Diagnosis: interpretation
The cause of spoilage is normally determined by reviewing the results from the investigational steps above and comparing them with the reported spoilage observations. This typically results in a solid understanding of what microorganism(s) were the immediate cause of spoilage and what intrinsic or extrinsic product factors enabled this spoilage to occur. There is no standard confirmation procedure. If the microbiological investigation leads to an isolated microorganism, it is possible to inoculate unspoiled product with that isolate, then package and incubate to mimic the conditions of the original spoiled product. If the observed spoilage condition is recreated in the inoculated product but not in an uninoculated control, that will provide strong evidence that we isolated the causative microorganism. However, if an inoculation study does not reproduce the observed spoilage, we cannot conclude that the microorganism isolated was not the causative agent – only that we do not fully understand all the circumstances. Considering the time needed for this additional step, it is more common to act without the information it may generate.
Determining the root cause
To this point, we’ve looked at factors that contribute to product shelf-life and approaches for identifying the immediate cause of product spoilage that might occur within the labeled shelf-life of the product. Sometimes, this stage of the investigation will reveal that the labeled shelf-life was unrealistically long for the product and the solution is to shorten the labeled shelf-life or modify some aspect(s) of the product and/or distribution system. With products that are relatively new to market, this may be because the initial product testing was not adequate to accurately establish a reliable shelf-life. With long-established products we sometimes see that multiple small changes have been made over an extended period and each had an intended beneficial effect: a cost saving, improved palatability through a small increase in product moisture content (raising aw), or an improved preference score through a small decrease in product acidity (raising pH). The net result of these changes is that the product drifts so far away from the conditions under which its shelf-life was established that it can no longer support the labeled shelf-life.
Other times, the root cause may be less obvious and require additional effort to evaluate steps in the sourcing, manufacture, and distribution of the product upstream of the point when the spoilage was observed. Those steps may not all be under the control of the same organization, hence our goal must be to learn enough to prevent the problem from reoccurring. This stage of the investigation may involve review of documents and specifications for indications that something unusual happened. It also may involve physically reviewing the upstream operations in sequence looking for sources of contamination, evidence of inadequate sanitation, signs of temperature abuse, etc. We should be aware that there may not be a single point of failure. Small adverse changes in several elements can happen inadvertently. Or a series of small but deliberate changes that individually have little impact can add up with premature spoilage as the unintended consequence.
Recovering the future
To prevent the spoilage condition from reoccurring, we must address the root cause(s) of failure identified in the investigation. If they are within our control, we can directly implement necessary corrective and preventive actions. When the root causes are out of our control, we must require that our suppliers take appropriate actions and, if that fails, be prepared to change suppliers. Then we must use appropriate controls to keep the operation in its new, more secure state.
A better way
Rather than experience the pain and cost of investigating the cause of a spoiled product and taking remedial action, it is better to take steps to design and make a product with the required shelf-life and then take further steps to manufacture and distribute it consistently in line with the design parameters.
Product design
We considered the intrinsic product factors and extrinsic environmental factors that determine product shelf-life in the section “Designing for shelf-life”. During product design, we need to balance the desired product sensory qualities with the intrinsic and extrinsic factors required to achieve our desired shelf-life. This can be a difficult task involving much trial and error. Experienced product developers have good intuition for how to approach this process efficiently and some modeling resources are available to help with parts of this process (5, 14, 15, 20). Note that models are not a substitute for expert knowledge or appropriate testing but are valuable for guiding study design and reducing product development time.
Challenge and stability testing
A prototype product can be deliberately inoculated with potentially important pathogens and/or spoilage microorganisms and evaluated over a period of storage in the intended distribution conditions and under “abuse” conditions. Periodic evaluation of the microbial population will show how long the product survives before significant changes occur. This microbiological challenge study allows us to understand what is likely to happen if some sanitary failure occurs during manufacture or packaging of the product.
A parallel sensory study can evaluate the appearance, smell, and taste of uninoculated prototype samples under the same storage conditions. At this stage of the product development cycle, we would want to do some pathogen testing of the prototype before tasting.
The results of these two studies can tell us what shelf-life to expect when the product is made and distributed under control, and when some element of the overall operation is out of control. They can also show us the most likely spoilage mode and help to estimate the pathogen risk.
A short list of questions in appendix 1 will help when preparing to engage a laboratory to help with challenge or shelf-life testing.
Specifications and verification testing
Once we have a product that delivers on all expectations for taste, texture, appearance and shelf-life, the key to avoiding future problems is to manufacture and distribute it consistently, meeting all of the design criteria. That is best done by following the principles of good manufacturing practice (3) and using appropriate routine testing to verify that all aspects of the operation are under control. Such testing could include simple and quick verification of product aw and pH and microbiological testing of both product and environmental samples for presence and levels of the most likely spoilage microorganisms. Specifications for acceptable results should be based on what can be achieved when the operation is under control. These tests can easily be added to the routine pathogen testing that is already being done. Guidance on microbiological specifications is described elsewhere (9).
Where to get help
Eurofins has many resources to help at all stages of product development and failure analysis.
- The National Food Laboratory can help with product design and
- Eurofins Assurance can help with developing specifications, standard operating procedures, training, and in-plant investigations.
- Eurofins Microbiology Laboratories in New Berlin, WI and Fresno, CA can help with the microbiological issues discussed in this whitepaper including spoilage diagnosis, challenge studies and shelf-life studies.
- The Eurofins Microbiology Laboratory based in Madison, WI, can assist with microbiome analysis using Next Generation Sequencing.
These resources can be accessed through a single point of contact with your local Eurofins laboratory or online at https://www.eurofinsus.com/food-testing/contact-us/
Acknowledgements
Thanks to Andrzej Benkowski, Dan DeMarco, and Chris Crowe for helpful comments on the manuscript.
References
- pH Values of Common Foods and Ingredients. Clemson Co-operative Extension. Food Systems and Safety. Available at: https://www.clemson.edu/extension/food/food2market/documents/ph_of_common_foods.pdf Accessed 06 October 2023.
- CABI Databases. Species Fungorum. https://www.speciesfungorum.org/Names/Names.asp Accessed 18 October, 2023.
- Code of Federal Regulations 21 CFR 110 Current Good Manufacturing Practice in Manufacturing, Packing, or Holding Human Food. Available at: https://www.ecfr.gov/current/title- 21/chapter-I/subchapter-B/part-110#110.5 Accessed 04 October 2023.
- Code of Federal Regulations 21 CFR § 113.3 Definitions. Available at https://ww.ecfr.gov/current/title-21/chapter-I/subchapter-B/part-113/subpart-A Accessed 24 August 2023.
- A Web Resource for Quantitative and Predictive Food Microbiology. https://www.combase.cc/index.php/en/ Accessed 19 October 2023.
- Dell ‘Amor, 2017. 100-Year-Old Fruitcake Found in Antarctica Is ‘Almost’ Edible. National Geographic. August 10, 2017. Available at: https://www.nationalgeographic.com/adventure/article/antarctica-fruitcake-scott-terra-nova Accessed 29 September 2023.
- Fontana , Anthony J. 2020. Appendix D: Minimum Water Activity Limits for Growth of Microorganisms. In Water Activity in Foods: Fundamentals and Applications, 2nd edition. Edited by G.V. Barbosa-Cánovas, A.J. Fontana Jr., S.J. Schmidt &, T.P. Labuza, pp. 571-572. Wiley, Available at: https://onlinelibrary.wiley.com/doi/full/10.1002/9780470376454.app4 Accessed 18 October 2023, 2023.
- Jarvis, G., C-K Hsu J.B. Pettengill, J. Ihrie, H. Karathia, N.A. Hasan & C.J. Grim. 2022. Microbiome Population Dynamics of Cold-Smoked Sockeye Salmon during Refrigerated Storage and after Culture Enrichment. Journal of Food Protection, 85, (2), 238–253.
- Legan, D. 2023. Microbiological Specifications in Food Operations. A Eurofins Whitepaper. Available at: https://www.eurofinsus.com/food-testing/resources/microbiological-specifications-in- food-operations/ Accessed 06 October 2023.
- Leistner, L. & Rödel, W. 1976. The stability of intermediate moisture foods with respect to micro- organisms. In Intermediate Moisture Foods. Davis, R., Birch, G.G., & Parker, K.J., eds. 120-137. Applied Science Publishers, London.
- Lewis, D. Arctic Explorers Uncover (and Eat) 60-Year-Old Food Stash. Smithsonian Magazine. October 22, 2015. Available at: https://www.smithsonianmag.com/smart-news/arctic- explorers-uncover-60-year-old-food-stash-180956936/ Accessed 29 September, 2023.
- Lund, M. & T. Eklund. 2000. Control of pH and use of organic acids. In The Microbiological Safety and Quality of Food, Vol 1 pp 175-199. Edited by B.M. Lund, T.C. Baird-Parker & G.W. Gould. Gaithersburg, MD. Aspen Publishers.
- M The Importance of Food pH in Commercial Canning Operations. Food Technology Fact Sheet FAPC 118. Robert M. Kerr Food & Agricultural Products Center. Oklahoma State University. Available at: https://extension.okstate.edu/fact-sheets/print-publications/fapc-food- and-agricultural-products-center/the-importance-of-food-ph-in-commercial-canning-operations- fapc-118.pdf Accessed 06 October 2023.
- Mullan, M.A. (2009). Predicting the safety and stability of products preserved with acetic acid using the CIMSCEE model. [On-line]. Updated 2013, 2022 Available at: https://www.dairyscience.info/index.php/food-model/177-cimscee-model.html Accessed: 19 October 2023.
- Mullan, M.A. (2015) Results of the mould free shelf life prediction. [On-line] Available from: https://www.dairyscience.info/newCalculators/mould1.asp Accessed: 19 October 2023.
- Reason, J. The Contribution of Latent Human Failures to the Breakdown of Complex Systems. Philosophical Transactions of the Royal Society of London. Series B, Biological Sciences 327:475-484.
- Reason, T., Carthey, J., & de Laval, M.R. 2001. Diagnosing “vulnerable system syndrome”: an essential prerequisite to effective risk management. Quality in Health Care, 10 (Suppl II): ii21– ii25.
- Schmidt, J. & A.J. Fontana. 2020. Appendix E. Water Activity Values of Select Food Ingredients and Products. In Water Activity in Foods: Fundamentals and Applications, 2nd edition. Edited by G.V. Barbosa-Cánovas, A.J. Fontana Jr., S.J. Schmidt &, T.P. Labuza, pp. 573-591. Wiley, Available at: https://onlinelibrary.wiley.com/doi/full/10.1002/9780470376454.app5 Accessed 18 October 2023.
- S Department of Agriculture. Food data Central Available at: https://fdc.nal.usda.gov/fdc- app.html#/ Accessed 18 October 2023.
- S. Department of Agriculture. Agricultural Research Service. Overview of Predictive Microbiology. Available at: https://portal.errc.ars.usda.gov/Overview.aspx Accessed 19 October 2023.
- S. Department of Agriculture Food Safety and Inspection Service. “Danger Zone” (40 °F – 140 °F). Available at: https://www.fsis.usda.gov/food-safety/safe-food-handling-and-preparation/food- safety-basics/danger-zone-40f- 140f#:~:text=%22Danger%20Zone%22%20%2840%20%C2%B0F%20-%20140%20%C2%B0F%29%201,40%20%C2%B0F.%20Place%20food%20in%20containers%20on%20ice. Accessed 18 October 2023
- S. Food & Drug Administration. 2022. Substances Added to Food (formerly EAFUS). Available at:https://www.cfsanappsexternal.fda.gov/scripts/fdcc/?set=FoodSubstances&sort=Sortterm_ID& order=ASC&startrow=1&type=basic&search=antimicrobial Accessed 18 October, 2022.
Appendices
When engaging with a laboratory during product development for shelf-life testing, or during a spoilage investigation, compiling answers to the questions in the following appendices will speed the process and aid in determining appropriate testing.
Appendix 1: Questions to consider when planning for a shelf-life or stability study.
- What is the Product? (Name and description)
- What is in it? Provide a complete ingredients list including Primary ingredients and preservatives. The concentration of any preservatives is also important.
- How is it made? Describe the process flow including intermediate steps such as mixing, intermediate hold times and temperatures, main process (baking, pasteurization, freezing, pressurization, hot fill, cold fill, etc.) and finishing processes.
- How is it packaged? (Packaging materials and processes, such as MAP or Vacuum Packing)
- What is the finished product state? (Ready to eat, par-cooked, raw, )
- How will it be distributed and stored condition? (Refrigerated, ambient, frozen, or other)
- List what you know about product
- pH
- Water activity
- Moisture
- Estimated shelf-life
- Target shelf-life
- Have you had a shelf-life analysis performed for this product or one similar? (Y/N)
- What is the goal for testing?
- Verification of current shelf-life
- New product – establishing shelf-life
- Change in ingredients, process, packaging
- R&D
- Other (Explain)
- Do you want to simulate ideal/recommended storage conditions via a real-time shelf-life study, or are you interested in an accelerated shelf-life study with modeling?
- Which stability parameters are you concerned about? (Microbiological, chemical, or organoleptic/sensory)
Appendix 2: Questions to consider when initiating a microbiological spoilage investigation.
- What is the Product? (Name and description)
- What “symptoms” of spoilage are you noticing?
- What is in it? Provide a complete ingredients list including Primary ingredients and preservatives. The concentration of any preservatives is also important.
- How is it made? Describe the process flow including intermediate steps such as mixing, intermediate hold times and temperatures, main process (baking, pasteurization, freezing, pressurization, hot fill, cold fill, etc.) and finishing processes.
- How is it packaged? (Packaging materials and processes, such as MAP or Vacuum Packing)
- What is the finished product state? (Ready to eat, par-cooked, raw, )
- How is it distributed and stored condition? (Refrigerated, ambient, frozen, or other)
- List what you know about product
- pH
- Water activity
- Moisture
- Estimated shelf-life
- Target shelf-life
- Is your product packaged at a co-man, or do you package on-site?
- Do you currently utilize an Environmental Monitoring Program?
- If yes, would you be willing to submit environmental monitoring samples to bolster data procured during the investigation of your product?
- Have you noticed anything unusual in your environmental monitoring results?
- Has anything changed recently (or shortly before the spoiled lot was made?)
The Authors:
J. David Legan, PhD
Scientific Director, Eurofins Microbiology Laboratories
Patricia Quinn
Analytical Services Manager, Eurofins Microbiology Laboratories